SCI. MAR., 60 (1): 89-97
SCIENTIA MARINA 1996 ADVANCES IN HYDROZOAN BIOLOGY, S. PIRAINO, F. BOERO, J. BOUILLON, P.F.S. CORNELIUS and J.M. GILI (eds.)
A. BRINCKMANN-VOSS
Department of Invertebrate Zoology, Royal Ontario Museum, 100 Queen’s Park, Toronto, Ontario M5S 2C6, Canada.
Mailing address: P. 0. Box 653, Sooke, British Columbia VOS 1NO, Canada
see this link for images of hydroids studied in this paper: https://www.racerocks.ca/tag/hydroid/
See this link for images of Tidepool # 6 “Anita’a Pool”
*Received November 29, 1994, Accepted October 20, 1995
SUMMARY: An assemblage of 27 hydroid species was reported from a tide pool in the lower rocky intertidal zone, and compared with 42 hydroids of the adjacent subtidal region. Location of hydroids within the pool, seasonal occurrence, growth and sexual maturity were tabulated, and some systematic aspects discussed . Possible causes of hydroid species diversity were considered, including location of the tide pool in an area of tidal rapids, and shading by surfgrass and rock cliffs during low tide.
Key words: tide pools, hydroids, seasonality, Pacific coast.
INTRODUCTION
Invertebrate species diversity is high around Race Rocks (48′ 18′ N, 123′ 32′ E), an archipelago in the Strait of Juan de Fuca between Vancouver Island, Canada, and Washington state, USA. (Fig.1,2). Although publications of marine invertebrates are available for the areas to the east and west of Race Rocks (Henkel, 1906; Fraser, 1913; Kozloff, 1983), information about invertebrates from Race Rocks is mostly limited to personal observations or unpublished reports: P. Breen, Pacific Biological Station Nanaimo, Dpt.Fisheries and Oceans; P. Lambert, curator of invertebrates, Royal British Columbia Museum; Garry Fletcher and student essays from Lester Pearson College of the Pacific: in Race Rocks Ecological reserves #97 publications list 1988-1994). Because of its rich biota, the area is now protected as an ecological reserve. Research on hydroids there, started in 1984, continues today by permit.
Taxonomic investigations have been published previously to characterize some of the hydroid species mainly from a tide pool on the west side of Great” (great is ommitted trom here on) Race Rocks (Brinck-mann-Voss 1988; Brinckmann-Voss et al., 1993) . However, information about the cornpositon and distribution of hydroid species in the pool is lacking, as is a comparison of its hydroid fauna with that of the surrounding intertidal and adjacent subtidal shelf.
The purpose of this paper is, therefore, to provide information on the hydroids of a cold temperate tide pool with regard to seasonal occurrence, growth and regression, and reproductive periodicities. This research is intended as essentially a faunal study rather than a dedicated ecological work employing methods and analysis such as described for rocky shores by Paine (1994).
DESCRIPTION OF STUDY AREA
The study area may be classified as protected outer coast, the main island of Race Rocks being protected by surrounding rocks and reefs . The west and north sides of the island are swept by very swift tidal rapids with a maximum velocity of 3 m /s (Fig.2). The average maximum velocity for the first half of June 1995 , which included a neap and leap tide, was calculated at 2.7 m/s (Canadian Tide and Current Tables, 1995).
The tide pool studied here is located on the west side of the island in the low intertidal zone in the middle of the Pseudobalanus cariosus – Mytilus californianus – Pollicipes polymerus belt (Lewis, 1964; Ricketts and Calvin, 1968; Carefoot, 1977). It may be classified as “Ic” after the pool classification summarized by Thomas (1983). The pool surface emerges at 1.5 m above 0 tide level (0 represents the chart datum in Canadian Tide and Current Tables) (Figs.3,5).
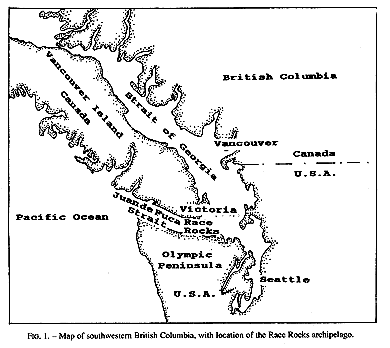
FIG. 1. Map of southwestern British Columbia,with location of the Race Rocks archipelago,
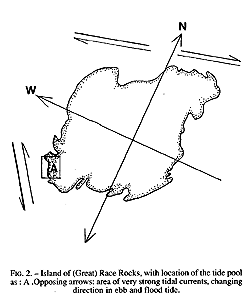
FiG. 2. – Island of (Great) Race Rocks, with location of the tide pool As: A .Opposing arrows: area of very strong tidal currents, changing direction in ebb and flood tide.
p89
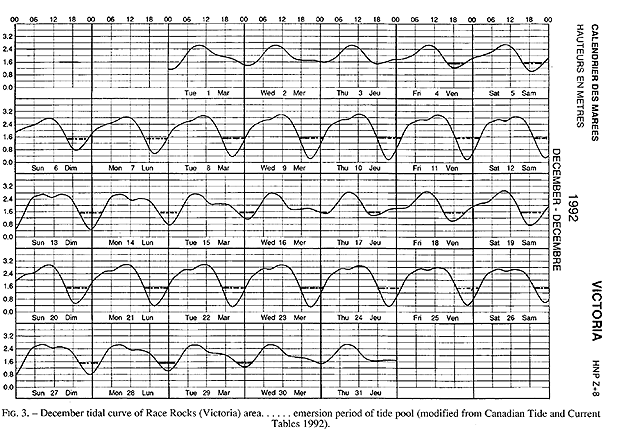
FIG. 3. – December tidal curve of Race Rocks (Victoria) area…… emersion period of tide pool (modified from Canadian Tide and Current Tables 1992).
Emersion of the pool surface is most obvious at leap tides. Although the pool may be exposed even at neap tides, waves and swells keep it awash, especially in stormy winter weather. During the emersion periods at leap tides, the pool does not drain and its water level changes are minimal. It is situated between a high cliff on the island side and a lower cliff toward the open sea. These two cliffs join at the north and south sides of the pool, thus forming a deep trough . Once emersed the pool is about 8m by 1.5m, slightly less wide in its northern part, with a maximum depth of 0.8m (Fig.4). It narrows in the middle with an exposed rock creating a small island (Fig.5). During incoming tide this is the first area to be flooded.
The surface temperature of the sea around Race Rocks varies during the year between 7 degrees C and 12 degrees C. Contrary to conditions in a shallow pool (without hydroids) 2m higher in the same area, surface and bottom temperatures in the lower and deeper pool, abundant with hydroids, scarcely differ from the sea temperature. Only during summer low tides, when the sun reaches the pool during the second third of the emersion period, are surface temperatures higher (2’C, rarely 42C )than the bottom temperatures or in the surrounding sea. Water temperatures were checked only February to October, because of the pool’s relative inaccessability in winter.
Unlike in the higher pool, salinities in the pool correspond with that of the adjacent sea (29-30%o, measurements provided partly by Garry Fletcher) from February to October.
No measurements of salinity and temperature were taken from November to January. However, the state of the surfgrass (Phyllospadix scouleri) lining the upper rim of the pool indicated that heavy winter rains in combination with low temperatures (Tokioka, 1963) impact the intertidal environment, including the rock pool. Lewis (1964), Carefoot (1977), and Thomas (1983) report on similar destructive effects of winter rains. The study area also encompassed the rocky infralittoral and subtidal, extending from 0-18m depth, to a distance of about 50 m from the shore around Race Rocks.
page 91
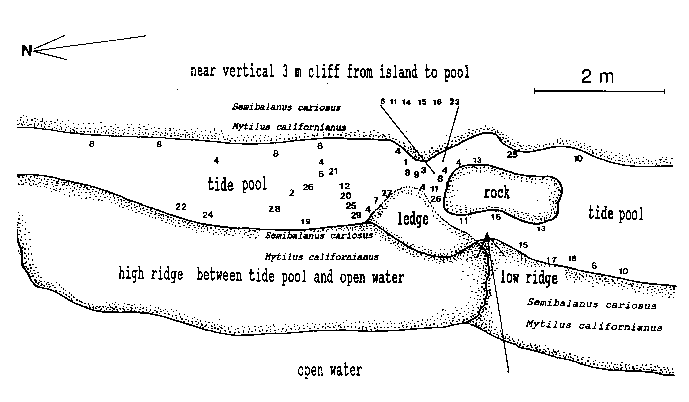
MATERIAL AND METHODS
Collections of hydroids from the tide pool were made from March to October, over a period from 1984 to 1994.
Hydroid specimens were returned live to the laboratory, where they were examined and some photographed. Many were cultured to establish their identity, especially when specimens were collected in the hydrorhiza stage only. Species, depth and location of the hydroids were mapped on a diagram on the site.
Samples from the sublittoral shelf were taken by divers. The samples that were collected included both hydroids and a variety of bottom material, such as kelp holdfasts, barnacles, and mussels. These substrates were examined for small hydroids in the laboratory.
RESULTS
Approximately 27 hydroid species were found in a tide pool in the intertidal zone, and 42 on the subtidal rocky shelf including the infralittoral fringe west and north of the island. Of these, 18 species are common in both their tide pool and subtidal area. The distribution of hydroids and seasonal occurrence in the tide pool are summarized in Fig.4 and tables 1-2. Hydroids from subtidal habitats including the infralittoral fringe are listed in Table 3.
92
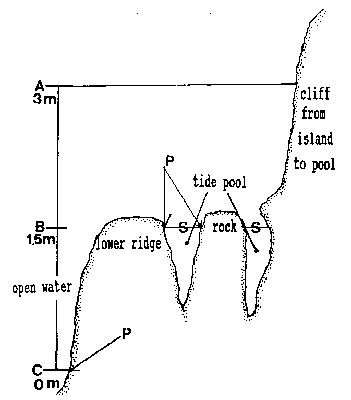
FiG. 5. – Cross-section of tide pool at area of rock in center of pool. A: High water of high spring tide. B: Emersion level of tide PWI. C: Lower low water of spring tide. A to C represent the intertidalregion. S: Surface of tide pool at 1.5 m tide level and lower tides. P: phyllospadix scouleir belt, located 1.5 m higher in the pool than on the open shore, where it can be found at the border between the intertidal and subtidal, marked as C
Except for one hydroid species (Rhizogeton nudus) among the stalks of Pollicipes polymerus, no hydroids were found in the intertidal outside the pool between pool and infralitioral fringe.
Species composition of the pool and of adjacent subtidal regions was similar, with a few notable exceptions: luxurious colonies ofSarsia eximia and Phialella sp. occurred on the rhizomes of Phyllospadix sculeri (Zosteraceae, Angiospermeae) lining the rim of the rock pool, but these species were less abundant in the Phyllospadix scouleri belt of the infralittoral of the open coast, (for location ofPhyllospadix scouleri see Fig.5). Conversely, larger species of the Sertulariidae were more diverse and abundant subtidally than in thepool. Plumularia setacea was common in the pool but less so subtidally, whereas Plumulara lagenifera was not found in the pool, but was abundant subtidally.
Hydroids of the tide pool were referrable to three groups. Group A showed marked seasonal cycles of activity and regression, with hydroid colonies represented during a dormant phase by hydrorhizae only. Species of group B were present periodically as small colonies, typically in early spring, and then enlarged during late spring and summer. Group C included species in which the activity patterns and colony sizes remained relatively unchanged throughout the year.
Included in group A was Hybocodon prolifer, abundant and fertile in late winter and spring, but reduced only to stolons in summer and fall. Sarsia eximia and Phialelia sp. (described by Boero 1987, but not named), living among the rhizomes of Phyllospadix scouleriplants (Zosteraceae), were reduced to hydrorhiza or to small colonies with a few sterile hydranths in late winter and spring. Both species occurred as large and fertile colonies in summer spreading occasionally onto the outer dead leaf sheadi of Phyllospadix or onto the surrounding rocks.
Peak reproduction was in June and July for Sarsia eximia and in September for Phialella sp.
Species of group B (e.g. Orthopyxis integral Obelia dichotoma, Garveia annulata, and Clavactinia sp.) were present as small patches in early spring. By late summer their colonies had enlarged and stolonal species covered wide areas on the rock walls of the pool. InClavactinia sp., reduction and expansion of the same colony was observed over a period of two years.
Species assigned to group C changed little in colony size during the year. These included representatives of the families Stylasteridae, Plumulariidae, and Sertulariidae. This may be related to their morphology, as their hydranths are more protected through the extensive perisarc of the colony, than species of group A and B.
DISCUSSION
Systematics: The taxonomy of hydroids from the northeastern Pacific, and especially of the Leptothecatae (terminology after Cornelius, 1992), is inadequately known. A large number of species has been reported from the area by Fraser (1913,1937), and before him by authors including Nutting (190015), Torrey (1902), and Clark (1876, 1877). However, many specimens collected from Race Rocks and adjacent areas do not correspond with descriptions of such species, a problem discussed by Brinckmann-Voss (1983) and Mills and Miller (1987). Detailed monographic revision is therefore needed from hydroids of the northeast Pacific. Comparisons are warranted with the hydroid fauna of the northwest Pacific (Yamada, 1959; Naumov, 1960; Antsulevich, 1992; Antsulevich and Vervoort, 1993), and with that of the circumpolar Arctic (Broch, 1909), as done by Kramp (1959, 1965, 1968) for the hydromedusae.
Accordingly, several species in this paper were identified only to the genus level (Tables 1-3). Work is currently underway on the Plumulariidae from Race Rocks and elsewhere on the British Columbia coast (Brinckmann-Voss and Calder, unpublish data). Changes of family and genus were made of two species of Anthoathecatac. Hataia parva Hirai and Yamada 1965, previously assigned to the Clavidae, is assigned here to the Acaulidae. Features described in the original (Hirai and Yamada, 1965) and in recent (Yamada and Kubota, 1989) work on the species as to its solitary nature, mode of asexual reproduction, and presence of stenoteles, justified placing Hataia near the genus Acaulis Stimpson, family Acaulidae. A species identified as Hydractinia milleriTorrey 1902 in Morris et al. (1980) is tentatively referred to the genus Clavactinia Thomely as Clavactinia sp. because its gastrozooids have several whorls of tentacles (Millard, 1975). The species listed in Morris, Abbott and Haderlie and found in the Race Rocks area is distinguished from H. milleri Torrey in
93
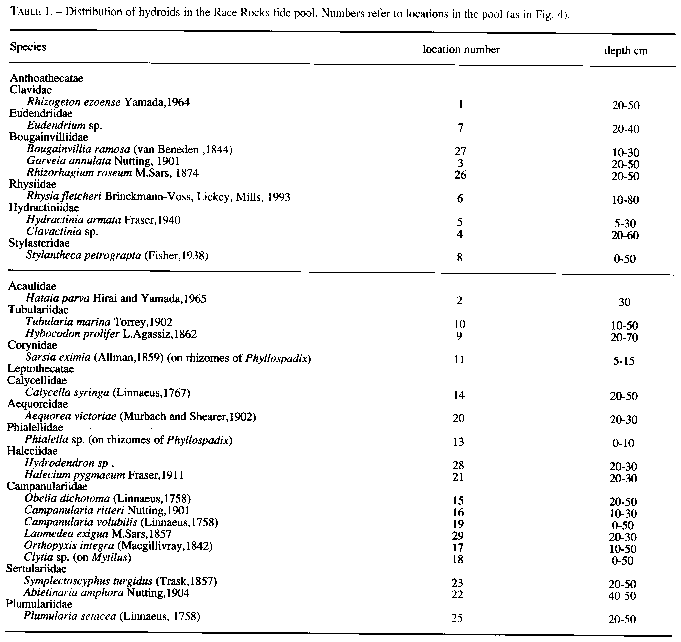
TABLE 1. Distribution of hydroids in the Race Rocks tide pool. Numbers refer to locations in the pool (as in Fig. 4).
Species
Anthoathecatae
Clavidae
Rhizogeton ezoense Yamada,1964
Eudendriidae
Eudendrium sp.
Bougainvilliidae
Bougainvillia ramosa (van Beneden , I 844)
Garveia annulata Nutting, 1901
Rhizorhagium roseum M.Sars, 1874
Rhysiidae
Rhysia fletcheri Brinckmann-Voss, Lickey, Mills, 1993
Hydractiniidae
Hydractinia armatata Fraser, 1940
Clavactinia sp.
Stylasteridae
Stylantheca petrograpta (Fisher, 1938)Acaulidae
Hataia parva Hirai and Yamada, 1 965
Tubulariidae
Tubularia marina Toney, 1902
Hybocodon prolifer L.Agassiz, 1 862
Corynidae
Sarsia eximia (Allman,1859) (on rhizomes of Phyllospadix)
Leptothecatae
Calycellidae
Calycella syringa (Linnaeus, 1767)
Aequoreidae
Aequorea victoriae (Murbach and Shearer, 1902)
Phialellidae
Phialella sp. (on rhizomes of Phyllospadix)
Haleciidae
Hydrodendron sp .Halecium pygmaeum Fraser, 1911
Campanularidae
Obelia dichotoma (Linnaeus, 1758)Campanularia ritteri Nutting, 1901
Canpanularia volubilis (Linnaeus,1758)
Laomedea exiguae M.Sars,1857
Orthopyxis integra (Mugillivray,1942)
Clytia sp. (on Mytilus)
Sertulariidae
Symplectoscyphus turgidus (Trask,1857)
Abietinaria amphora Nutting, 1904
Plumulariidae
Plumularia setacea (Linnaeus, 1758)
having several purple eggs per gonophore, in lacking spines on the colony, and in having two types of gastrozooids. Torrey (1902) originally described H. milleri from Monterey Bay, California, and reported only one orange egg per gonophore. Mills and Miller (1978) also reported Hydractinia milleri with one egg. Clavactinia sp. is common on rock walls in the tide pool, and on rockwalls and overhangs in sheltered spots exposed during tides below the 0 level or chart datum (in Canadian tide and current tables 1984-1995). A description of this species is in preparation, together with a revision of the Hydractiniidae of the British Columbia coast (Brinckmann-Voss, unpublished data).
. Ecological remarks:
Although tide pools have
94
been studied frequently in different parts Of the world, little research has been published about them. Most work on tide pools has involved studies of algae and their reaction to changing salinity, temperature, desiccation, sometimes in comparison to open waters (Doty, 1957; Lewis, 1964; Carefoot, 1977; Newell, 1979; Thomas, 1983). More detailed investigation on fauna and flora of tidepools was reported by Stephenson et al. (1934), Pyefinch (1943), and Emson (1986), but there is very little information on hydroids in such pools. This is probably because the hydroid fauna of the intertidat zones – except for the infralittoral fringe (Stephenson and Stephenson, 1972; Carefoot, 1977; Kozloff, 1983; Calder, 1991 a,b) – is rather limited, as papers
on intertidal zonation show (e.g. Southward, 1958; Ricketts and Calvin, 1968).
Cornelius (1988) listed the hydroid fauna of tide pools at Holme next the Sea (U.K.), but in that case the hydroids were swept in from offshore areas, and were not autochthonous to the pool.
Some of the references cited above may help explain the diversity and abundance of hydroids in the Race Rocks tide pool:
1. Doty (1957) mentioned the abundance of organisms at the rim of tide pools, probably related to the “edge effect” reported by other ecologists (Carefoot, 1977). It may be assumed that the luxurious colonies of Phialella sp. and Sarsia eximia in the root system of the surfgrass immediately below the water surface of the pool could be related to
this edge effect, although its cause may be difficult to pinpoint.
2. Wedler (1975), studying comparable sites, reported of a greater abundance of hydroids in shaded than in sunny areas , where algae tend to dominate. (The same was observed by the author on the shaded and sunny side of floating docks). The qualitative and quantitative abundance of hydroids and relatively few algae in the pool may be influenced by this “shade ” effect, because the pool is shaded during emersion by the leaves of the surfgrass which form a canopy on its surface.
3. Lewis (1964) reported the influence of different velocities of tidal rapids on the intertidal fauna. From that work it may be inferred that the tidal rapids sweeping the tide pool area of Race Rocks
TABLE 2. – Seasonality of hydroids in the Race Rocks tide pool, March to October. Smaller and less abundant hydroid species, difficult to detect without taking material out of the pool, are marked (?); species with living hydranths absent were marked (-); those with hydranths present (+); those with gonads < colonies augmenting in size, > diminishing in size. For authors see table I
Species M A M J J A S O
Anthoathecatae
Clavidae
Rhizogeton ezoe se – – + – ++ ++ + +
Eudendriidae
Eudendrium sp. + + + + + + + +
Bougainvilliidae
Bougainvillia ramosa + + + + + + + +
Garveia annulata + +< ++ ++ ++ ++ ++ ++
Rhizorhagium roseum + + ++ ++ + ? ? ?
Rhysiidae
Rhysiafletcheri + ++ ++ ++ ++ ++ ++ ++
Hydractiniidae
Hydractinia armata – + < ++ ++ ++ ++ ++ ++
Clavactinia sp. + + ++ ++ ++ ++ ++ ++
Stylasteriidae
Stylantheca petrograpta + + + + + + + +
Acaulidae
Hataia parva ? ? ? + ? ? ? ?
Tubulariidae
Tubularia marina – – ++ ++ ++ ++ ++ ++
Hybocodon prolifer ++ ++ + – – – – –
Corynidae
Sarsia eximia + ++ ++ ++ >+ + + +
Leptothecatae
Calycellidae
Calycella syringe ? ? ++ ++ ++ ++ + +
Aequoreidae
Aequorea victoriae Phialellidae? ? ? ? + ? ? ?
Phiatella sp. < ++ ++ ++ ++ ++ ++ >
Haleciidae
Halecium pymaeum + + + ++ ++ ++ ++ ++
Hydrodendron sp. + + + + + + + +
Campanulariidae
Obelia dichotoma + <++ <++ ++ ++ ++ ++ ++
Campanularia ritteri + + ++ ++ ++ + + +
Campanularia volubilis + ++ ++ ++ ++ ++ ++ ++
Orthopyxis integra + <+ <++ <++ ++ ++ ++ ++
Laomedea exigua ? ? ? ? ? ++ ? ?
Clytia sp. ? ? ? + ++ ++ ++ ++
Sertulariidae
Symplectoscyphus turgidu s + + ++ ++ ++ ++ ++ ++
Abietinaria amphora ++ ++ ++ ++ ++ ++ ++ ++
Plumulariidae
Plumularia setacea + ++ ++ ++ ++ ++ ++ ++
95
TABLE 3. – Hydroids identified from the infralittoral fringe and subtidal areas of Race Rocks, to a depth of 18 m, mostly west and north of the tide pool. Asterisks indicate species which were also found in the tide pool.
Anthoathecatae
Clavidae
Rhizogeton nematophorum Antsulevich, 1986Rhizogeton ezoense* Yamada, 1964
Rhizogeton nudus Broch, 1910
Eudendriidae
Eudendrium sp. (probably not same species as in tide pool) Bougainvilliidae
Bougainvillia sp. (not ramosa)
Garveia annulata* Nutting, 1901
Rhizorhagium roseum* M.Sars, 1864
Rhysiidae
Rhysiafletcheri* Brinckmann-Voss, Lickey and Mills, 1993 Hydractiniidae
Hydractinia armata* Fraser, 1940Hydractinia laevispina Fraser, 1922
Clavactinia sp. *
Stylasteridae
Stylantheca petrograpta* (Fisher, 1938)Stylaster venustus (Verrill, 1870)
Tubulariidae
Tubularia marina* Torrey, 1902Tubularia sp. (less than 12 aboral tentacles)
Corynidae
Coryne crassa Fraser, 1914Sarsia eximia* (Allman, 1859)
Sarsiaproducta (Wright, 1858)
Sarsia tubulosa (M.Sars, 1835)
Leptothecatae
Calycellidae
Calycella syringa* (Linnaeus, 1767)Filellum sp. (?parasiticum Antsulevich 1987)
Haleciidae
Hydrodendron gracile (Fraser, 1914)Halecium pygmaeum* Fraser, 191 1
Lafoeidae
Hebella sp.Campanulariidae
Campanularia ritteri* Nutting, 1901Campanularia volubilis (Linnaeus, 1758)
Campanularia sp.
Clytia sp.
Obelia dichotoma* (Linnaeus, 1758)
Orthopyxis Integra
Sertulariidae
Abietinaria abietina (Linnaeus, 1758)Abietinaria amphora* Nutting, 1904
Abietinaria greenei (Murray, 1860)
Abietinaria anguina (Trask, 1857)
Hydrallmania distans Nutting, 1899
Symplectoscyphus turgidus* (Trask, 1857)
Symplectoscyphus sp. tricuspidatus? (Alder, 1856)
Thuiaria sp.
Aglaopheniidae
Aglaophenia inconspicua Torrey, 1904Aglaophenia latirostris Nutting, 1900
Plumulariidae
Plumularia setacea*(Linnaeus, 1758)
Plumularia lagenifera Allman, 1885
Kirchenpaueriidae
Kirchenpaueria plumularoides (Clark, 1876)
play an important role in the establishment of the hydroid fauna there.
Work on seasonality of hydroids has been done in different marine environments and climates (Riedl, 1959; Bouillon, 1975; Wedler, 1975; Boero and Fresi, 1986; Brinckmann-Voss, 1987; Calder, 1990; Garcia-Rubies, 1987,1992). Seasonal changes in the Race Rocks tide pool are most evident from late fall to spring – mainly on the species near the surface, when a marked regression occurs in numerous species. This regression is most likely caused by dilution of surface salinities during rain storms in the low winter tides (Carefoot, 1977; Thomas, 1983).
ACKNOWLEDGEMENTS
Research for this paper would not have been possible without the help of Garry Fletcher (senior biologist), Theo Dombrowsky and students of the Lester Pearson College of the Pacific (Metchosin, B.C., Canada). I thank them and the administration of the College for their help. Garry Fletcher introduced me to the tide pool on Race Rocks, instructed his students in searches for hydroids by diving, and made numerous collections by diving himself. Joan and Charles Redhead, former lighthouse keepers at Race Rocks, allowed me to stay at their lighthouse residence for several days during low tide periods, which allowed me to go back to the tide pool and check details during the same low tide period. I acknowledge their hospitality and help. Dale Calder (Royal Ontario Museum, Toronto, Canada) helped with this paper from its early stages through numerous discussions, advice and reviews, for which I am very thankful. I thank Paul Cornelius ( Natural History Museum, London, U.K.) for all help, especially with search for ecological literature, and I thank Stephen Cairns (National Museum of Natural History, Washington, U.S.A.) for identifying the Stylasteridae.
96
PLEASE NOTE: THIS VERSION HAS BEEN SCANNED BY OPTICAL CHARACTER RECOGNITION. MOST HAS BEEN CORRECTED BUT IT MAY STILL HAVE A FEW ERRORS. -GF
REFERENCES
Antsulevich, A. – 1992. Observations on the hydroid fauna of the Kurile Islands. Sci. Mar. 56(2-3):213-216.
Antsulevich, A. and W. Vervoort. – 1993. Some little known species of hydroids (Cnidaria: Hydrozoa: Lafoeidae) and description ofPapillionella pterophora gen. nov. spec. nov. Zool. Meded. 67: 431-443.
Boero, F. 1987. – Life cycles of Phialella zappai n.sp., Phialella fragilis and Phialella sp. (Cnidaria, Leptomedusae,Phialellidae) from central California. J. nat. Hist. 21: 265-480.
Boero, F. and E.Fresi. – 1986. Zonation and evolution of a rocky bottom hydroid community. P.S.N.I.-Mar. Ecol. 7:123-150.
Bouillon, J. – 1975. Sur la reproduction et 1’6cologie de Paracoryne huvei Picard (Tubularoidea-Athecata-Hydrozoa- Cnidaria). Arch. Biol., 86:45-96.
Brinckrnann-Voss, A.- 1983. British Columbia marine faunistic report on the Hydrozoa, part 2 Hydroids. Canadian Technical Rep.of Fish. Aquat Sci, 1185: 1-20.
Brinckmann-Voss, A. – 1987. Seasonal distribution of hydromedusa (Cnidaria, Hydrozoa) from the Gulf of Naples and vicinity, with observation on sexual and asexual reproduction in some species. In: J. Bouillon, T. Boero, F. Cicogna and P.F.S. Cornelius (eds.)Modern Trends in the Systemtics, Ecology, and Evolution of Hydroids and Hydromedusae, pp. 133-141. Clarendon Press, Oxford.
Brinckmann-Voss, A. – 1988. Sarsia cliffordi n.sp. (Cnidaria, Hydfozoa, Anthomedusn) from British Columbia with distribution records and evaluation of related species. Can. J. Zool. 67: 685-691.
Brinckmann-Voss, A., D.M. Lickey, and CF. Mills. – 1993. Rhysia fletcheri (Cnidaria, Hydrozoa, Rhysiidae) a new species of colonial hydroids from Vancouver Island (British Columbia, Canada) and the San Juan Archipelago (Washington, U.S.A.). Can. J. Zool. 71: 401-406.
Broch, H. – 1909. Die Hydroiden der arktischen Meere. Faun Arctica 5: 129-247.
Calder, DR. – 1990 . Seasonal cycles of activity and inactivity to some hydroids from Virginia and South Carolina, U.S.A. Can.J. Zool.68: 442-450.
Calder, DR. – 1991 a. Associations between hydroid species assemblages and substrate types in the mangal at Twin Cays, Belize. Can. J. Zool. 69:2067-2074.
Calder,D.R. 1991 b.Vertical zonation of the hydroid Dynamena crisioides ( Hydrozoa, Sertulaiiiidae) in a mangrove ecosystem at Twin Cays,.Belize. Can. J. Zool. 69: 2993 2999.
Canadian Tide and Current Tables, Volume 5, Juan de Fuca Strait and Strait of Georgia.- 1984-95. Dpt. Fish. Oceans, Ottawa, Canada.
Carefoot, T. – 1977. Pacific Seashores. J.J. Douglas Ltd, Vancouver.
Clark, S. F. – 1876. The hydroids of the Pacific coast of the United States, south of Vancouver Island. With a report on on those in the museum of Yale College. Trans Connecticut Acad Arts Set., 3: 249-264.
Clark, S. F. – 1877. Repon on the hydroids collected on the coast of Alaska and the Aleutian Islands, by W. H. Dall, U. S. Coast Survey, and party, from 1871 to 1874 inclusive Proc. Acad. Nat. Sci. Philadelphia 1876: 209-238.
Cornelius, P.F.S. 1988. Hydroid collection from intertidal pools at Holme next the Sea, Norfolk. Trans. Norfolk and Norwich Field Soc., 28: 72 78.
Cornelius, P.F.S. – 1992. Medusa loss in leptolid Hydrozoa (Cnidaria), hydmid rafting, and abbreviated life-cycles among their mmote-island faunae: an interim review. Sci. Mar. 56: 245-261.
Doty, M.S. – 1957. Rocky Intertidal Surfaces. Geol.Soc. Ame. Mem. 67: 535-585.
Emson,R.H. 1986.Life history patterns in rock pool animals.in: P.G.Moore and R.Seed (eds), The Ecology of Rocky Coasts. pp. 220-222. Columbia University Press, New York.
Fraser, C.M. – 1913. Hydroids from Vancouver Island and Nova Scotia. Bull. Victoria Mem. Mus. :147-188.
Fraser,C.M. 1937.Hydroids of thePacificCoast ofCanada and the United States. University of Toronto Press, Tomnto.
Garcia Rubies, A. – 1987. Distribution of epiphytic hydroids on Posidonia sea gmss. In: J. Bouillon, T. Boero , F. Cicogna and P.F.S. Cornelius (eds.) Modern Trends in the Systematics, Ecology, and Evolution of Hydroids and Hydromedusae, pp. 143-155. Clarendon Press, Oxford.
Garcia Rubies, A. – 1992. Habitat differentiation among epiphytic hydroids of the smgms Posidonia Oeanica from the Medes islands (NE Catalonia, Spain). Sci.Mar. 56: 263-267.
Henkel, I. – 1906. A study of tide-pools on the west coast of Vancouver Island. Postelsia 2:277-304.
Hirai E. and M. Yamada. – 1965. On a new athecate hydroid Hataia parva – n. gen., n. so. Bull. Mar. Biol. Stn. Asamuchi, .12: 59-62.
Kozloff, EN. – 1983. Seashore Life of the northern Pacific coast, rev. edition. Douglas and McIntyre, Vancouver.
Kramp, P.L. – 1959. The Hydromedusn of the Atlantic Ocem and Adjacent Waters. Dana Rep. 46: 1-283.
Kramp, P.L. – 1965. The Hydmmedusae of the Pacific and Indian Oceans, 1. Dana Rep. 68: 1-162.
Kramp, P.L. – 1968. The Hy&medusae of the Pacific and Indian Oceans, 11, III. Dana Rep. 72: 1-200.
Lewis, JR. – 1964. The Ecology of the rocky shores. ‘The English University Press, London.
Millard, N.A.H. – 1975. Monograph of the Hydroida of Southern Africa. Ann. S. Afri. Mus. 68: 1-513.
Mills, C. and R. Miller. – 1987. Hyroid polyps. In: Kozloff, E.N. (ed), Marine Invertebrates of the Pacific Northwest. pp. 44-62 Univ. Washington Press, Seattle.
Morris, R.H., Abbott, D.P, and Haderlie, E.C. – 1980. Intertidal Invertebrates of California. Stanford University Press, Stanford, California.
Naumov, D.V. 1960. Hydroids and Hydrowdusae of the USSR pan I-VI. English translation of original Russian in Israel Program for Scientific Translation , Jerusalem 1969 cat. no. 5108.
Newell, RC. – 1979. Biology of Intertidal Animals. Marine Ecological Surveys Ltd., Famshm, Kent.
Nutting, C.C. – 1900-1915. American hydroids, part 1-3. Smithsonian Institution, United States National Museum, Special Bulletin.
Paine, R.T. – 1994. Marine Rocky Shores and Commnity Ecology: An Experiwntalist’s Perspective. Excellence in Ecology:4. Ecology Institue, Oldendort/Luhe.
Pyefinch, K.A. – 1943.The interlidal ecology of Bardsey Island, North Wales , with special reference to the m-colonisation of rock surfaces, and the rock pool environment. J. Anim.Ecol. 12:82 108
Race Rocks Ecological Reserves #97. Publications List. B.C Parks, Ecological Reserves Office, 800-2 Johnsen Street, Victoria, B.C. V8V I X4 Canada.
Ricketts, E.F. and J. Calvin. 1968. Between Pacific Tides, 4th. ed., revised by J.W. Hedgpeth. Stanford Univ. Press, Stanford.
Riedl,R. – 1959. Die Hydroiden des Golfes von Neapel und ihr Anteil and der Fauna unterseeischer H6hlen. Ergebnisse der osterreichischen Tyrrhenia Expedition 1952 Teil 16. Pubbl. Stn. Zool. Napoli. 30 ( Suppl.) :589-755.
Southward, A.J. – 1958. The Zonation of Plants and Animals m Rocky Sea Shares. Biol-R”., Cambridge Phil. Soc. 33: 137-177.
Stephenson, T.A. and A. Stephenson. – 1972. Life between Tidemarks on Rocky Shores. W.H. Freeman and Company, San Francisco.
Stephenson,T.A., Zmnd, A. and J. Eym. – 1934.The liberation and ufilisation of oxygen by the population of rock pools. J. Exp.Biol. II: 162-172.
Thomas, M.L.H. – 1983. Marine and coastal systems of the Quoddy region, New Bmnswick, Canada. Can. Spe. Publ. Fish. Aqua.Sci. 64: 1-306.
Tokioka,T. – 1963. Supposed effects of the cold weather of the winter 1962-63 upon the intertidal fauna of the vicinity of Seto.Publ. Seto Mar. Lab. 9(2) :415-437.
Torrey, H.B. – 1902. The Hydroids of the Pacific Coast of North America with special reference to the species in the collection of the University of California. Univ. Calif. Public. Zool. 1: 1-104.
Wedler, E. – 1975. Okologische Untmuchungen an Hydroiden des Felslitorals von Santa Marta (Kolumbien). Heigolinder wiss.Meeresunters. 27: 324-363.
Yamada, M. – 1959. Hydroid fauna of Japan and its adjacent water. Publ. Akkeshi Mar. Biol. Star. 9: 1-101.
97